Background of quorum sensing and acyl homoserine lactones
Explanation of the sensing circuit in E. coli and in B. subtilis
Collected results and discussion of our laboratory work
Sensing circuit
We established a system for detection and eradication of pathogens using genetically engineered Bacillus subtilis cells. Upon detecting a specific quorum sensing (QS) molecule produced by a certain pathogen, our B. subtilis sleeper cells produce and release phages specific for the invading pathogen. We realized that with a sensing circuit to control the phage induction (Figure 1). Here, genes needed for phage induction are controlled with an inducible promoter. In our specific case, the induction of the lambda phage is regulated by a protein called RecA7301,2, as explained in more detail in the chapter phage induction. Therefore, we regulate the expression of this protein with our system. In this manner, our sensing circuit is responsible for the controlled induction of phages by our sleeper cell when pathogens are present. This makes the circuit crucial for the protection of the biofilm against invading pathogens.
The inspiration for our sensing system was drawn by a paper released early in 2021. Here, Pseudomonas aeruginosa was detected using its own quorum sensing system implemented in another organism. The paper realized this in E. coli cells which expressed egfp as a reporter upon detection of the QS molecule of P. aeruginosa.3 We adapted this system for the usage in B. subtilis and instead of egfp inserted recA730 as target gene to be expressed when P. aeruginosa is present.
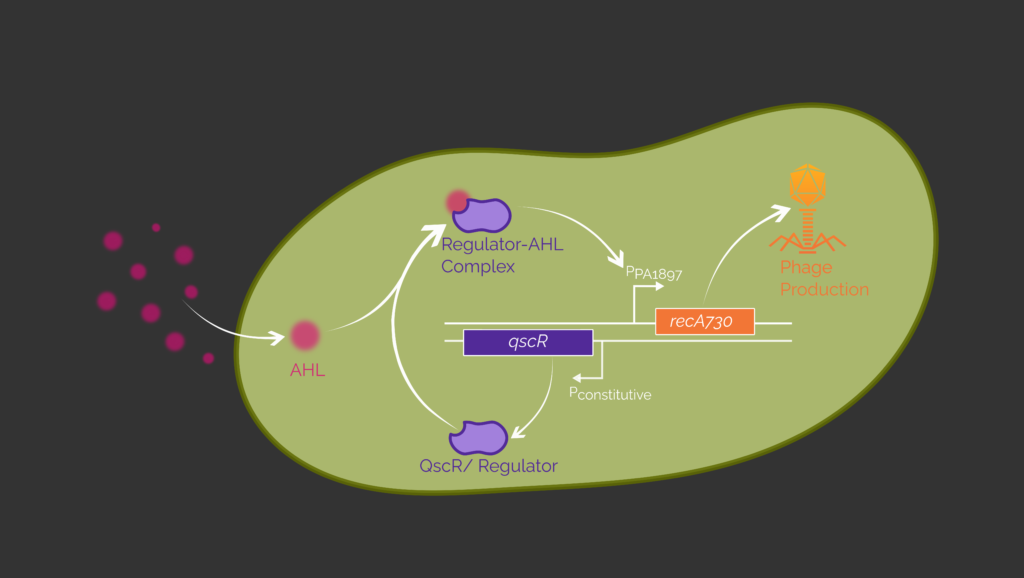
In our genetic circuit, the detection of pathogens and the expression of recA730 as a response are divided into two transcriptional units (Figure 1). In the first unit, the transcription factor QscR originating from P. aeruginosa is expressed constitutively. The second transcriptional unit includes the respective promoter which is inducible by the QscR-AHL complex. The transcription of the protein RecA730 is controlled by this inducible promoter.
In our design, QscR will be produced independently of the presence of the pathogen. If there are P. aeruginosa cells nearby the B. subtilis cells, its QS molecule N-(3-oxododecanoyl)-homoserine lactone (3OC12-HSL), will diffuse into the B. subtilis cell and bind to QscR. The QscR-3OC12-HSL complex can interact with the second promoter PPA1897, which will then enable the production of RecA730.
Saeidi et al. (2011) also used the QS system for the detection of pathogens. But instead of QscR, LasR was chosen as a transcription factor. The difference between both is that LasR can not only detect 3OC12-HSL but other similar AHL as well.5 Also, it forms dimers before binding to its associated promoter PLux.6 Apart from that the system is the same, which is why we want to test both variants.
In summary, our goal is to detect as small amounts of 3OC12-HSL as possible, which will then lead to an activation of the sleeper cell. Our activated phage defence system will then release specific phages against the pathogen. This results in lysis of the pathogen. In this way, we use the pathogens own QS system against it.
Design of the Sensing Circuit in E. coli
Wu et al. (2021) and Saeidi et al. (2011) have already detected P. aeruginosa successfully in E. coli with both transcription factors, QscR and LasR, using eGFP as a fluorescence output.3,5 For the design of our genetic circuit, we therefore decided to start by reproducing their results before transferring the circuit to B. subtilis.
The genetic circuits (Figure 2) consists of two transcriptional units (TUs). The first unit encodes for the allosteric transcription factor (aTF), which is constitutively expressed. If present, 3OC12-HSL binds to the aTF. They form a complex, which binds the promoter in the second transcriptional unit. This induces the expression of egfp, which allows us to measure the output of the induction.
As shown in Figure 3 the transcription factor as well as the related inducible promoters are variable parts. Both coding sequences are codon optimized for E. coli. We will use the T7 promoter as the constitutive promoter in TU 1 like Wu et al. (2021) and Saeidi et al. (2011). For the T7 promoter, we need an E. coli strain containing a T7 polymerase. For this purpose, we use E. coli BL21 (DE3).3,5
Additionally, we will exchange the aTF with the fluorescent protein mKATE2 to test the function of the constitutive promoter. This way, we can quantify the gene expression on the basis of the fluorescence output, allowing us to approximate the amount of aTF that would be constitutively expressed. At the same time, we test the basal expression of the inducible promoters PPA1987 and PLux by measuring the eGFP output in the absence of transcription factor. The basal expression is needed for the model of the genetic circuit.
We use the T7 terminator and an E. coli consensus RBS which was already used by Wu et al. (2021).
Design of the Sensing Circuit in B. subtilis
After we demonstrated the function of the genetic circuit in E. coli, we can transfer it to B. subtilis. Until now a genetic circuit that is able to detect P. aeruginosa by its quorum sensing molecule 3OC12-HSL has not been shown in B. subtilis. Therefore, we plan to use a Design-Build-Test-Learn (DBTL) cycle to create and optimize such a genetic circuit. This way, we aim to find the most sensitive and reliable genetic circuit to detect P. aeruginosa in B. subtilis.
To optimize the genetic circuit, we want to combinatorially test the two transcription factors LasR and QscR, their respective promoters, the ribosome binding sites of TU 1, as well as the strength of the constitutive promoter in TU 1 (Figure 4). Using a fluorescent protein as circuit readout, allows us to identify the best combination of the tested parts within the circuit. In the following section, we explain the parts of interest in greater detail.
We want to compare both transcription factors to see which one works best in B. subtilis. Therefore, we codon optimized the sequences encoding the two transcription factors for B. subtilis and ordered them together with the sequences of the two respective promoters. Note that LasR and QscR control PLux and PPA1897, respectively.3,5
We decided to test three constitutive promoters of different expression strengths to find the ideal amount of transcript and thus translated aTF to trigger the expression of egfp. All constitutive promoters originate from B. subtilis and are listed in the iGEM parts registry as part of the Bacillus Biobrick Box of the iGEM Team of LMU-Munich from 2012. According to the experiments of the iGEM Team LMU 2012, the three promoters order the following from weak to strong: PliaG < PlepA < Pveg. We will use mKATE2 as a reporter protein to test the function of the promoters.
We planned to test different ribosome binding sites (RBS) to improve protein translation: First a B. subtilis consensus RBS from the iGEM parts registry, and second a RBS sequences calculated by the Salis lab RBS calculator7 for optimal translation initiation of the proteins QscR and LasR. For the expression of egfp we just use the B. subtilis consensus RBS. We will combinatorially screen the RBS and promoter sequences to optimize mKATE2 output.
We use the B. subtilis rrnB terminator in both transcription units as recommended to us by Prof. Dr. Jan-Willem Veening.
After we generate the reliable genetic circuit in B. subtilis, we can exchange egfp with recA730 and combine the sensing circuit with the genetic switch by which phages change from lysogenic into lytic cycle .
References
- 1. Chapter One: The master elements of control. In: A Genetic Switch: Phage Lambda Revisited. 3rd ed. Cold Spring Harbor (NY): Cold Spring Harbor Laboratory Press; 2004.
- 2. Vlašić I, Šimatović A, Brčić-Kostić K. Genetic Requirements for High Constitutive SOS Expression in recA730 Mutants of Escherichia coli. Journal of Bacteriology. 2011:4643–4651. http://dx.doi.org/10.1128/jb.00368-11. doi:10.1128/jb.00368-11
- 3. Wu Y, Wang C-W, Wang D, Wei N. A Whole-Cell Biosensor for Point-of-Care Detection of Waterborne Bacterial Pathogens. ACS Synthetic Biology. 2021 Jan 26:333–344. http://dx.doi.org/10.1021/acssynbio.0c00491. doi:10.1021/acssynbio.0c00491
- 4. Lequette Y, Lee J-H, Ledgham F, Lazdunski A, Greenberg EP. A Distinct QscR Regulon in the Pseudomonas aeruginosa Quorum-Sensing Circuit. Journal of Bacteriology. 2006:3365–3370. http://dx.doi.org/10.1128/JB.188.9.3365-3370.2006. doi:10.1128/jb.188.9.3365-3370.2006
- 5. Saeidi N, Wong CK, Lo T, Nguyen HX, Ling H, Leong SSJ, Poh CL, Chang MW. Engineering microbes to sense and eradicate Pseudomonas aeruginosa, a human pathogen. Molecular Systems Biology. 2011 Jan:521. http://dx.doi.org/10.1038/msb.2011.55. doi:10.1038/msb.2011.55
- 6. O’Reilly MC, Dong S-H, Rossi FM, Karlen KM, Kumar RS, Nair SK, Blackwell HE. Structural and Biochemical Studies of Non-native Agonists of the LasR Quorum-Sensing Receptor Reveal an L3 Loop “Out” Conformation for LasR. Cell Chemical Biology. 2018 Sep:1128-1139.e3. http://dx.doi.org/10.1016/j.chembiol.2018.06.007. doi:10.1016/j.chembiol.2018.06.007
- 7. Design Ribosome Binding Site Sequences to Control Protein Expression Levels. De Novo DNA. RBS Calculator. 2021 [accessed 2021 Jul 7]. https://salislab.net/software/design_rbs_calculator